SLS Block 1 missions: Returning to the Moon using only the basic SLS
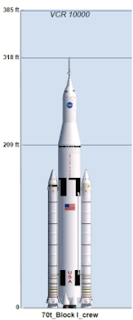
In my previous blog, I wrote about canceling the Block 2 SLS because I considered it unnecessary, claiming that Block 1 should be more than enough. Now, to be fair, Block 1 will probably not last us long enough for a serious program because the current booster casings only allow up to 10 flights. After some time, Block 1 will run out of boosters and we'll have to make new ones, which would basically turn it into Block 1A with advanced boosters. Still, I think that the initial 90 and 105 metric ton capacities of these vehicles are more than enough, and in this article I will describe a possible lunar mission using only Block 1 capabilities. Block 1 capabilities The Block 1 payload to Low Earth Orbit is usually described as 70 metric tons. However, if you have paid close attention to the project and have an eye for rocketry, you might have noticed that this isn't true. Initially, SLS was to have four different variants: Block 0, Block 1, Block 2 and Block 3. Block 0 w...